Radiation therapy for locally advanced lung cancer
Definitive RT
RT alone
Definitive radiotherapy as a therapeutic strategy for LANSCLC is based on the landmark study reported by Roswit et al. in 1966 (1). This randomized control trial (RCT) found RT doses of 40 to 50 Gy using 1.75 to 2 Gy per fraction resulted in an overall survival (OS) benefit when compared to observation (1-year OS 18.2% vs. 13.9%, respectively). While OS was limited, local control as a means of improving overall outcomes was established. Further improvement in survival was sought in the first dose escalation conducted by the RTOG. Here, RTOG 73-01 compared four radiation fractionation schemes. This included a split course regimen of 40 Gy, as well as 40, 50, and 60 Gy delivered at 2 Gy per fraction, 5 fractions per week (2). While the split course had the lowest survival (2-year OS 10%), the other dose regimens were equivalent in regards to survival (2-year OS 45%). Maturation of the data demonstrated that the 60 Gy arm, when compared to the other conventional treatments of 50 Gy and 40 Gy had higher intrathoracic control [3-year local regional control (LRC) 67% vs. 58% vs. 56%, respectively] (3). This dose response relationship was supported by additional RTOG Trials where dose was found to correlate with an increase OS (4). Consequently, 60 Gy at 2 Gy per fraction became the standard of care for LANSCLC.
Hyperfractionation benefits
Seeking to improve upon the results of standard fractionation, various fractionation schemes were developed in an attempt to improve local control. This included hyperfractionation which is the delivery of higher doses in the same overall treatment time but with more fractions (e.g., 2 fractions per day). Total dose must be increased to account for the lower dose per fraction to achieve equivalent local control. When hyperfractionation is applied clinically, typically two fractions are delivered per day (with a 6-hour interfraction interval to allow for normal tissue repair). For example, RTOG 8311 was a phase I/II dose escalation trial using hyperfractionation. Dose was escalated using 60, 64.8, 69.9, 74.4, and 79.2 Gy at 1.2 Gy twice daily (BID) fractions (5). This feasibility study demonstrated that hyperfractionation as a means of escalating dose did not lead to a significant increase in acute or late effects on normal tissue. Also, the 69.6 Gy arm showed an improved OS in a subset of patients with stage III disease [American Joint Committee on Cancer (AJCC) 1984], ≤5% weight loss, and Karnofsky performance scale (KPS) ≥70. Hyperfractionation can also be delivered using a three fraction per day approach referred to as continuous hyperfractionated accelerated radiotherapy (CHART). CHART was explored in a RCT where 60 Gy in 2 Gy daily fractions was compared to 54 Gy in 1.5 Gy fractions given three times a day (TID) (6). The TID regimen was associated with an OS advantage, predominantly in squamous cell carcinoma histology, while again showing no difference in late dysphagia and moderate/severe pneumonitis. A follow-up dose escalation study (CHARTWEL) compared 60 Gy using 1.5 Gy TID fractions against 54 Gy using 1.5 Gy TID fractions (7). The high dose arm was associated with higher acute esophagitis and late mild pulmonary morbidity. Additionally, ARO97-1 compared the CHARTWEL regimen against 66 Gy using 2 Gy daily fractions (8). While CHARTWEL may have improved local control in those with advanced disease, when including all cohorts the OS, local control, and rate of distant metastasis were the same.
Sequential chemoradiation therapy
Concurrent with the development of hyperfractionation regimens, the introduction of chemotherapy to the management of LANSCLC occurred. The CALGB were the first to demonstrate that induction cisplatin and vinblastine followed by 60 Gy (2.0 Gy per fraction) improved median survival for inoperable LANSCLC when compared to RT alone (13.7 vs. 9.6 months, respectively) (9). Additionally, the benefit of sequential chemoradiotherapy (CRT) was demonstrated in the Intergroup (INT) study as induction chemotherapy followed by 60 Gy in 2 Gy fractions led to an increase in median survival when compared to RT alone using 60 Gy in 2 Gy fractions and altered fractionation RT alone using 69.6 Gy with 1.2 Gy BID fractions (13.8 vs. 11.4 vs. 12.3 months, respectively) (10). These two trials established induction chemotherapy followed by 60.0 Gy at 2.0 Gy per fraction as the standard management for LANSCLC prior to the development of concurrent CRT.
Concurrent chemoradiation therapy
Though the CALGB and INT trials established sequential CRT over RT alone as the standard of care for LANSCLC, multiple concurrent CRT trials established this to be superior over sequential therapy as seen in Table 1. A Japanese trial included 312 patients with unresectable stage III NSCLC and compared concurrent CRT (split course RT) to sequential therapy (11). The median survival and OS was improved in the concurrent CRT arm at the expensive of increased myelosuppression. Additionally, RTOG 9410 performed a 3-arm study in patients with unresectable stage II-IIIB (99% were stage III) disease (12). Here, sequential CRT using conventional fractionation to 63 Gy, concurrent CRT using conventional fractionation to 63 Gy, or concurrent chemotherapy using hyperfractionation, 69.6 Gy at 1.2 Gy BID were compared. After a median follow-up of 11 years, the concurrent CRT arm was reported superior to that of sequential therapy by demonstrating an OS benefit of 6%. Several other studies contributed to the establishment of concurrent CRT to be superior to sequential chemotherapy using radiotherapy doses between 60 to 66 Gy (12-14) (Table 1). In 2010, the NSCLC Collaborative Group published a meta-analysis analyzing sequential and concurrent CRT trials concluding that concurrent CRT provides an OS benefit (5.7% at 3 years; 4.5% at 5 years) nevertheless at the cost of increased acute grade 3 or 4 esophageal toxicity (18% vs. 4%) (15).
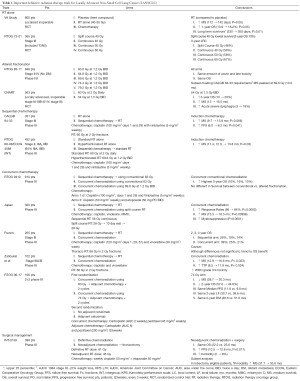
Full table
Multiple chemotherapeutic agents have been delivered concurrently with radiotherapy however platinum based dual agents are standard with carboplatin and paclitaxel often favored over cisplatin and etoposide given the lower toxicity profile (16,17). Additionally, dosing has varied from weekly to full dose every-3-weeks (Q3weekly) regimens. Belani et al. randomized 404 patients with LANSCLC treated with definitive concurrent CRT to either carboplatin with weekly paclitaxel or Q3weekly paclitaxel and carboplatin (18). Both arms had similar median survivals and time to progression. The weekly paclitaxel arm had more grade 3 or 4 anemia and the Q3weekly arm had more neuropathy and arthralgia. The authors concluded both treatment strategies were acceptable.
However, given the low survival after definitive management, consolidative chemotherapy was studied to improve these outcomes. The Southwest Oncology Group (SWOG) phase II S9504 trial demonstrated promising results using consolidative docetaxel after concurrent chemoradiation for stage IIIB patients (19). The results showed a 3-year OS of 37% and a median survival of 26 months. However, the Hoosier Oncology Group conducted phase III trial evaluating the use of consolidative docetaxel after concurrent chemoradiation therapy and no survival difference was seen (20). Therefore, while consolidative chemotherapy is often given after concurrent chemoradiation therapy for stage III patients, there is no randomized evidence to support routine use. A recent meta-analysis, however, did show maintenance chemotherapy for advanced non-small lung cancer may increase progressive free survival and OS (21).
Surgical management for locally advanced NSCLC
While definitive CRT is standard for the majority of LANSCLC, surgery remains an option for a limited subset of Stage IIIA disease. The INT-0139 trial compared neoadjuvant concurrent CRT using 45 Gy in 1.8 Gy fractions followed by surgical resection versus definitive CRT therapy alone (22). The final results showed no difference in OS between both groups. However, an unplanned subset analysis suggested that those who were eligible for a lobectomy (versus pneumonectomy) had an improvement in median survival (33.6 vs. 21.7 months). Additionally, a French study analyzed 702 patients with resected N2 disease and stratified based on clinical staging, single nodal involvement, or multi-station nodal involvement (23). The authors reported that single station, microscopic N2 disease had the highest 5 year OS (34%) while clinically positive, multistation N2 disease had limited outcomes (3%). From these series, surgical management is favored for stage IIIA patients with low volume, single nodal station disease, that are eligible for a lobectomy prior to the initiation of systemic therapy. In terms of neoadjuvant treatment, the radiotherapy dose should be between 45 and 54 Gy (24).
Dose escalation
Efforts to improve OS in the setting of definitive concurrent CRT have focused on dose escalation as a means of improving local control and subsequent survival. In 2001, a phase I/II trial for dose escalation with concurrent chemotherapy in unresectable stage IIIA/B NSCLC demonstrated the feasibility of dose escalation from 60 to 74 Gy with concurrent and induction carboplatin and paclitaxel with only 8% grade 3-4 toxicity (25). In 2004, a second phase I dose escalation trial with concurrent chemotherapy for unresectable stage III NSCLC evaluated doses from 78 to 90 Gy (26). Here, dose escalation to 90 Gy was achieved without dose-limiting toxicity and grade 3 esophagitis occurred in only 16%. These data lead to a recent dose escalation trial using concurrent CRT followed by consolidative chemotherapy. Specifically, RTOG 0617 trial was a 2×2 study evaluating 60 Gy against 74 Gy and the addition of consolidative cetuximab along with concurrent and adjuvant carboplatin and paclitaxel (27). The study was powered to detect a median survival benefit of 7 months. Surprisingly, the study was halted after an interim analysis reported the 74 Gy provided no benefit in terms of survival and potentially was detrimental.
Several explanations for the poor survival in the 74 Gy arm have been postulated (28,29). Interestingly, the inferior survival could not be accounted for by treatment-related deaths, local control, or distant metastasis between the high dose and low dose arms. There may have been uncaptured grade 5 deaths at community sites. Discrepancies in causes of mortality on death certificates and autopsy reports have been reported in the literature, and can happen in up to 47% of cases (30). This theory is supported by the heart V5 and V30 being linked to OS on multivariate analysis. Moreover, heart and lung dose constraints were suggested but not enforced and only half of the centers used intensity modulated radiation therapy (IMRT). For centers using IMRT, poor dose calculations and variable heart contours could have affected dosimetric outcomes. The high dose arm had poorer heart contours than the low dose arm (28). Perhaps requiring proper dose constraints for critical organs at risk, necessitating use of IMRT, and providing standard organ contours could have produced different results.
Movsas et al. reported on patient quality of life (QOL) in RTOG 0617 (31). QOL was measured using a Physical Well Being, Functional Well Being, and Lung Cancer Subscale index. While the final results showed no difference in treatment related morbidity, patients in the 74 Gy had a significant lower QOL at 3 months than the 60 Gy arm. The authors also reported baseline QOL was a predictor for survival. However, IMRT was associated with a higher QOL over 3D-CRT. These findings lead the authors to conclude IMRT may improve the therapeutic window for LANSCLC.
The choice of chemotherapy regimens could have influenced the survival outcomes as well. The increase in mortality in the high dose group commenced within 3 months of being randomized. During this period, patients would be receiving consolidative paclitaxel and carboplatin. It is known sequential taxanes after radiotherapy increases toxicity including pneumonitis (32). This is supported by the fact less patients completed consolidative chemotherapy in the high dose arm. Perhaps different chemotherapy regimens should be used in the adjuvant setting.
Finally, the longer treatment time using conventional fractionation may have contributed to the survival difference in the high dose arm. It is known longer treatment times may lead to poor survival for advanced NSCLC patients (33). The longer treatment time could allow for tumor re-population. In CHART, 54 Gy given in 1.5 Gy BID fractions (2.5 weeks) provided a survival advantage over 60 Gy in 2 Gy fractions (6 weeks) (6). The shorter treatment time accounted for tumor re-population. Shorter treatment times with equal dose equivalence via use of hypofractionation may overcome this concern.
While RTOG 0617 did not show a benefit for high dose radiation, other factors could have contributed to the final results. Therefore, the concept of dose escalation should not be abandoned especially given the rapid advances in RT including IMRT, 4D-CT simulation scans, motion gating, image guided therapy, adaptive RT, and use of hypofractionation. Utilizing these techniques in addition to stricter protocol requirements in the setting of dose escalation, and alternative adjuvant chemotherapy options, may provide more favorable results.
Concluding remarks and ASTRO guidelines
Therefore the ideal radiotherapy dose with concurrent chemotherapy for LANSCLC is between 60 Gy to 66 Gy with no randomized benefit seen above 60 Gy. Higher doses close to 74 Gy are associated with inferior outcomes. If concurrent CRT cannot be delivered consideration of sequential therapy or RT alone can be advocated, of which altered fractionation may be an option. If not, 60 Gy using 2 Gy fractions is the most appropriate regimen. If the patient is lobectomy eligible, a dose of 45 to 54 Gy with concurrent chemotherapy is acceptable in a select subset. The ASTRO guideline statements conclude that the standard RT given with concurrent chemoradiation therapy is 60 Gy in 2 Gy daily fractions over 6 weeks (34). If RT alone is utilized, a minimum dose of conventional fractionated 60 Gy is recommended to optimize local control. Altered fractionation has been explored with RT alone and has a strong recommendation. A summary of the landmark studies is provided in Table 1.
Radiation therapy techniques
CT-based treatment planning
Prior to the advent of computerized tomography (CT), 2-D lung treatment planning was performed using planar radiographs to define the field boundaries and dose calculation was performed in a single plane using rough measurements of the patient’s body contour. This dose calculation also ignored tissue density changes in the lung.
Three-dimensional treatment planning based on CT scans enabled more accurate definition of target volumes and more accurate dose calculation accounting for tissue heterogeneity. In CT-based treatment planning, the gross tumor volume (GTV) is outlined, and a margin is added to include suspected microscopic spread of disease, creating the clinical target volume (CTV). To obtain the planning target volume (PTV), an additional margin is added to account for setup error and intrafraction tumor movement. Three-dimensional planning also allows detailed evaluation of doses received by tumor targets and by adjacent organs using Dose Volume Histograms (DVHs). Both institutional as well as a SEER analysis suggest that 3D treatment planning improves survival (35,36).
Volume delineation with CT-based treatment planning
When treating nodal areas, we have progressed from using Elective Nodal Radiotherapy (ENRT) to involved field irradiation (IFI). ENRT was used given the risk of microscopic disease harboring in the neighboring hilar and mediastinal nodal eras. Previous surgical series have shown occult mediastinal metastasis can be found in 20% of clinically node negative patients (37). However, treating elective nodal areas leads to larger treatment volumes which increases the risk of normal tissue toxicity. Also, there was published data in which 524 patients with NSCLC treated with IFI using 3D conformal RT had a 2-year elective nodal control of 92.4%. This control was likely due to incidental radiation eradicating subclinical microscopic disease as discussed below (38).
Yuan et al. addressed whether ENRT is equivalent to IFI in a RCT in which 200 inoperable stage III NSCLC patients treated with concurrent CRT were randomized to receive ENRT or IFI (39). Patients receiving IFI had higher local control, higher response rates, and decreased pneumonitis, but were treated to higher doses. The out of field recurrence rates were equivalent between both groups. This led to the prevalent adoption of treating involved nodal groups only.
In regards to adequately covering microscopic disease from the primary tumor, the histology determines the extent of CTV. Surgical series have shown local microscopic extension is larger for adenocarcinoma than squamous cell carcinoma (40,41). Given these differences a margin of 8 mm is suggested for adenocarcinoma and 6 mm for squamous cell carcinoma.
Targeting PET/CT
The advent of the 18F-fluorodeoxyglucose (FDG) positron emission tomography–computed tomography (PET-CT) has greatly assisted in target delineation. PET-CT scans are superior to CT or PET alone for detection of mediastinal nodal metastasis (42). When compared to conventional CT scans, PET has increased sensitivity from 61% to 85% and increased specificity from 79% to 90% in regards to detection of lymph node metastasis (43). Registration of PET-CT scans to the treatment simulation CT has also led to greater consistency for defining the GTV (44).This can allow for IFI to be more confidently delivered. The PET-START trial was the first RCT to compare PET-CT treatment planning to standard treatment CT planning (45). Results included an increase in the amount of stage IV patients identified and a trend in OS for those who received combined CRT therapy in the PET-CT group.
While PET-CT has these advantages, it has limitations seen in high false positive results for clinically node positive patients and thus should not be used to replace surgical mediastinal staging (46). There is currently an open phase II trial utilizing PET-CT for adaptive RT which will be discussed later.
Motion management
Respiratory movement has always been a major concern in thoracic irradiation (47). Unfortunately, tumor movement takes place throughout the respiratory cycle. This leads to the possibility of the tumor missing significant amounts to dose throughout the course of treatment. It also leads to artifact formation in CT scanning resulting in difficulty contouring the GTV. Due to these concerns, proper motion management techniques are recommended for movement greater than 5 mm in any dimension.
Historically, tumor motion was accounted for by adding a margin around the CTV to create the internal target volume (ITV). This becomes challenging for tumors with significant respiratory motion, such as those near the diaphragm, where superior-inferior motion can be more than 3 cm (48). The additional ITV leads to a large treatment volume which increases the risk of normal tissue toxicity and limits the ability for dose escalation. However, motion management accounts for tumor motion which allows for dose escalation without the added risk of increased toxicity (49). Several methods for motion management exist but can be broadly categorized into respiratory gating or tumor tracking techniques. We will discuss respiratory gating first and then tumor tracking techniques.
Many respiratory gating techniques utilize four dimensional CT (4D-CT) scans. This process involves a simulation CT scan during which multiple images (typically 10-12) are obtained throughout the respiratory cycle at each axial slice (50). The abdominal motion, as a surrogate for the respiratory cycle, is recorded concurrently during this process for appropriate temporal correlation. This surrogate motion may be recorded, for example, by a camera system measuring the motion of a reflective marker on the patient’s abdomen, or by measuring pressure changes in a belt placed around the patient’s abdomen. The signal from the abdominal surrogate is then used to bin the CT images, resulting in series of separate CT scans for each phase in the breathing cycle. These scans can then be viewed in a movie loop to show how the tumor moves.
The appropriate phase window for treatment, one in which the total tumor motion is limited to a defined threshold (for example, total motion ≤5 mm) is identified. The treatment window is typically near the end of exhalation, since this tends to be the longest and most reproducible part of the breathing cycle. During treatment, an equivalent abdominal surrogate signal is used to control the beam on time of the linear accelerator. Choosing a narrower phase window will produce a tighter limit on tumor motion, but it will also lengthen treatment.
Abdominal compression may also be used to decrease the amount of diaphragmatic motion which in turn reduces respiratory tumor motion. The compression technique has the advantage that the treatment beam on time is not limited to just part of the breathing cycle, so overall treatment times may be shorter than those for gating. However, compression may be uncomfortable for patients, particularly those whose respiration is already compromised.
Another respiratory motion management technique is active breath control (ABC). In this procedure, the patient breathes through a digital spirometer which is connected to a balloon valve (51). The system can suspend the patients breathing at a specified lung volume, typically at deep or moderate inhalation (52,53). After taking a few preparatory breaths, the patient is asked to breathe in to a fixed volume indicated by a video display. The valve is then closed for a patient-dependent period (typically 15-30 seconds), during which irradiation of the tumor takes place.
Another means of accommodating respiratory motion is to reposition the radiation beam dynamically so as to follow the tumor’s changing position, referred to as real-time tumor tracking (54,55). Real-time tumor tracking can be achieved by using a dynamic MLC or a linear accelerator attached to a robotic arm (55). This technique requires continuous monitoring of the position of the tumor (or surrogate), which may be accomplished by tracking fiducial markers or direct fluoroscopic imaging of the tumor.
Regardless of the method, motion management is a promising technique which can allow for appropriate dose delivery to the actual tumor site while sparing critical organs at risk. Figure 1 shows an example of the importance for motion management.
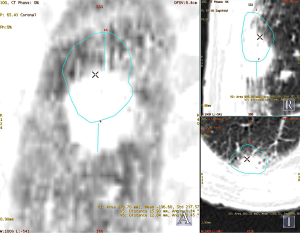
IMRT
IMRT is gaining popularity in the treatment for various malignancies (56). This technique results in increased conformity and greater sparing of normal tissue than three dimensional conformal radiation therapy (3D-CRT) (57,58). This allows for decreased rates of treatment related toxicity. Yom et al. published a retrospective review of advanced NSCLC treated with CRT comparing those treated with IMRT against 3D-CRT. IMRT resulted in reduced levels of grade 3+ pneumonitis. Liao et al. previously published a retrospective review comparing IMRT against 3D-CRT (59). Lower rates of grade 3 or higher pneumonitis were reported in the IMRT group which was thought to be secondary to a lower lung V20 value. While the V20 was higher in the 3D-CRT group, the V5 was higher in the IMRT group. These data suggest that IMRT is associated with reduced treatment related morbidity. This in return can lead to higher rates of treatment compliance. This coincides with an ASTRO Abstract published on QOL in the dose escalation RTOG 0617 study (31). This secondary analysis evaluated patient reported outcomes and its effect on survival. Interestingly, while no significant difference in toxicity between the high dose and low dose arm was found, lower patient reported QOL was more prevalent in the high dose arm at 3 months and was associated with a decrease in survival. IMRT use was also associated with less QOL decline than 3D-CRT. This gives grounds for future phase III trials evaluating IMRT vs. 3D-CRT in the treatment for LANSCLC.
However there are concerns for IMRT delivery. IMRT has steep dose gradients potentially risking decreased coverage for a moving target. This disadvantage can be accounted for by gating technology as discussed above. Second, IMRT leads to more low dose spillage. Lastly, the more accurate target definition may provide a potential disadvantage. While 3D-CRT fields are conformed to the target, other mediastinal lymph nodes not contoured but in the path of the beam will receive a significant dose (60). This incidental irradiation is suggested to eradicate subclinical microscopic metastasis regional nodal stations (38). This suggested benefit in 3D-CRT may be lost with highly conformal irradiation with steeper dose gradients. Figure 2 shows a dosimetric comparison of 3D-CRT and IMRT for a LANSCLC patient.
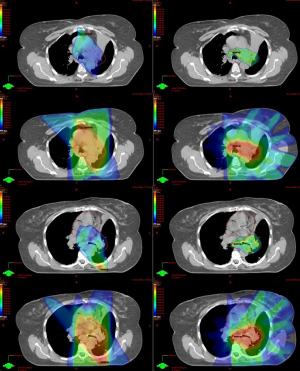
Organs at risk
Maintaining proper dose constraints for the lung, esophagus, and heart are critical to decrease treatment related morbidity associated with CRT. Graham et al. showed the lung volume receiving 20 Gy (V20 Gy) was a predictor for radiation pneumonitis and grade 2 or higher can occur in 36% of patients if the V20 Gy exceeds 40% (61). Other reports show a reduction in radiation pneumonitis when V5 Gy ≤60%, V10 ≤50%, V30 ≤18 Gy and mean lung dose (MLD) is less than 17 Gy using conventional fractionation (62-65). We therefore recommend keeping the MLD <17 Gy, V5 ≤60%, V10 ≤40%, V20 <30% to keep the grade 2 or higher toxicity to less than 11%.
Additionally, excessive esophageal dose leads to increased morbidity and mortality. Singh et al. evaluated predictors for radiation induced esophageal toxicity in patients with NSCLC. He reported 58 Gy was the threshold dose for acute grade 3-5 esophageal toxicity for those who received concurrent CRT (66). Takeda et al. also showed the volume receiving greater than 35 Gy was a predictor for acute toxicity for both NSCLC and small cell lung cancer patients treated with thoracic radiation (67). The literature suggests esophageal volumes receiving above 40 to 50 Gy correlates with acute symptoms and the prescription dose should be the maximum dose allowed to the esophagus (68).
Heart constraints are also important. In 2010, a SEER analysis reported on over 34,000 patients treated with chemotherapy and/or radiation for NSCLC between 1991 to 2002 (69). The large retrospective review identified an association with CRT treatment and the development of ischemic heart disease, cardiomyopathy, and cardiac dysfunction. Left sided tumors treated with radiation therapy alone were associated with an increased risk of heart failure. While there is a paucity of data regarding heart dose constraints for patients treated specifically for LANSCLC, the recent RTOG 0617 trial showed the V5 and V30 heart constraints influenced survival (27). In this trial, the recommended constraints were V60 Gy <33%, V45 Gy <66%, and V40 Gy <100%. However, adequate heart dose constraints will need to be defined and confirmed to assure the best therapeutic ratio for LANSCLC. Acceptable heart dose constraints are V30 Gy ≤50% and V45 Gy ≤35% to reduce the risk of pericarditis (70).
Future of definitive radiation therapy
Adaptive radiation therapy
Despite multidisciplinary advances for lung cancer management, local control and survival remain low. Escalating the radiotherapy dose may improve local control, however, there are limitations given the need to respect normal tissue toxicity. One method of combating this dilemma is through the emerging technique of adaptive radiation therapy. In this technique, a PET-CT is obtained during the treatment course. The initial course of radiation therapy uses the GTV identified on initial staging scans. However, a repeat PET-CT is obtained after a defined dose and the cone down dose is delivered to the residual FDG avid volumes. University of Michigan conducted a pilot study in 2007 to assess whether tumor and lung metabolic response during treatment correlated with post-treatment responses using PET-CT scans (71). After 45 Gy, 73% of the patients had a partial response and 13% had a complete response. The qualitative response after 45 Gy correlated with the overall response after radiation. The same group had a follow-up prospective study evaluating the use of dose escalation in adaptive planning showing adaptive RT allowed for a significant reduction in treatment volumes and allowed for dose escalation with a range of 30-102 Gy (mean 58 Gy) to be safely administered (72).
RTOG 0116 is a going randomized phase II trial evaluating Adaptive Radiation Therapy using a FDG PET-CT scan during treatment. This trial consists of stage IIIA and IIIB NSCLC patients. The control arm will receive 50 Gy in 2 Gy fractions, receive a FDG PET-CT scan, and then continue therapy to 60 Gy. The experimental arm will receive 46.2 Gy using 2.2 Gy fractions, receive a FDG PET-CT scan, and then receive adaptive radiotherapy based on the new PET metabolic tumor volume up to a total dose of 80.4 Gy.
Hypofractionation
NSCLC cells have a cell doubling time of approximately 3 days and accelerated repopulation during radiation therapy is well described (73). Each additional daily treatment after 6 weeks of treatment is associated with a 1.6% decrease in survival (74). This concept is so important that it’s postulated to be one of the reasons the high dose arm had a poorer survival in the RTOG 0617 trial. Hypofractionated radiation therapy may provide a valuable option to overcome this hurdle. Using this technique an equivalent or higher biological equivalent dose (BED) may be given in an equal or shorter treatment time. While there are concerns with organs at risk with this technique, hypofractionation has been shown to safely and effectively allow for dose escalation when given with or without concurrent chemotherapy for advanced stage NSCLC (73,75). The fraction dose given with chemotherapy has ranged from 2.4 to 3.0 Gy (73).
The EORTC phase I/II hypofractionation trial used 2.75 Gy fractions to a dose range of 60.5 to 66 Gy with concurrent cisplatin (76). The majority of these patients were advanced stage. This feasibility study showed low rates of both acute and late toxicity. The 2-year local disease-free interval rate was 58%. The follow up EORTC Trial which compared sequential versus concurrent chemotherapy with hypofractionated radiotherapy also had promising results (77). This trial used 2.75 Gy fractions. The authors reported low rates of both acute grade 3 or 4 hematologic toxicity and esophagitis and low rates of late grade 3 or 4 pneumonitis and esophagitis. Both of studies consisted predominately of advanced staged patients and used single agent chemotherapy. However, the SOCCAR Trial used dual agent chemotherapy and only consisted of stage III NSCLC (78). This phase II trial used 2.5 Gy per fraction up to 55 Gy and randomized between sequential and concurrent chemotherapy. Early results show treatment related mortality to be 2.9%, grade 3 or higher esophagitis to be 8.8%, and the 2-year OS to be 50% in the concurrent chemotherapy arm.
We know from previous trials, hyperfractionation using BID or TID allows for successfully dose escalation with a survival benefit. However, hypofractionation has the benefit of providing these same advantages while being more convenient for the patient. It is also important to note, given the higher dose per fraction, conformal techniques such as IMRT, motion management, and image guided therapy should be employed to ensure our best efforts of decreasing normal tissue toxicity.
Palliative
Patients with stage IV NSCLC are often treated with palliative radiotherapy to alleviate symptomatic burden. Indications for this form of therapy include dyspnea, bronchial obstruction, hemoptysis, superior vena cava syndrome, and pain (34,79).
Different dose schedules have been evaluated in multiple randomized controls; each showing hypofractionated radiation therapy can provide adequate palliation (80-82). Common dose schedules used are 30 Gy in 10 fractions,20 Gy in 5 fractions, 17 Gy in 2 weekly fractions, and 10 Gy in 1 fraction (34). Higher dose regimens are associated with higher rates of symptomatic improvement, more prolonged palliation, and a modest improvement in survival principally with those with a good performance status (34,83,84). However, this is at a cost of increased toxicity such as esophagitis. More succinct fractionation schedules (e.g., 20 Gy in 5 fractions, 17 Gy in 2 weekly fractions, and 10 Gy in 1 fraction) have also shown to provide adequate relief with decreased rates of toxicity (34). These shorter schedules also have an added benefit of shorter delays to chemotherapy and thus can be more efficiently assimilated between cycles.
Endobronchial brachytherapy has also been evaluated and reviewed as palliative treatment for NSCLC. This technique has the advantage of delivering high dose irradiation to a localized luminal tumor through a catheter. There is no standard dose/fractionation regimen although a range from a single fraction of 10 to 15 Gy to quadruple fractions of 3.8 Gy has been reported (85-87). Endobronchial brachytherapy is able to deliver a higher dose per fraction with a more rapid dose falloff. This aspect of treatment has a theoretical advantage of allowing for higher rates of symptomatic improvement with lower rates of normal tissue toxicity. Interestingly however, a 2006 Cochrane meta-analysis showed external beam radiation therapy (EBRT) is superior to brachytherapy for initial palliation and there is no additional advantage to combined modality (88). However endobronchial brachytherapy remains a valuable treatment option for those who have progressed through prior palliative EBRT, irradiation needed for a previously irradiated area (whether definitive or palliative), or lung obstruction in a non-metastatic patient with attempts to expand the lung for definitive treatment (34).
Concurrent chemotherapy for palliative external beam radiation therapy
Palliative radiation therapy has the benefit of providing relief in a shorter period of time than chemotherapy. Given systemic chemotherapy combined with radiation therapy has improved outcomes for those with LANSCLC, the question arises if the same is true for those needing radiation therapy for palliative intent. There are several studies evaluating the feasibility and outcomes of this question, albeit, with variations in systemic agents, radiation schedules/doses, and patient factors (89-91). However, there is an Australian RCT designed to specifically answer this question. After randomizing 200 patients to palliative radiation therapy with or without chemotherapy, the authors concluded the addition of chemotherapy resulted in a higher radiographic response rate with no improvement in palliation, OS, or disease free survival (92). There was also a significant increase in toxicity for combined modality. The study is limited, however, in that an uncommon chemotherapy for LANSCLC was given (fluorouracil), radiographic response was measured by plain radiographs, and patients received a high dose per fraction (4 Gy × 5 fractions).
Therefore the question of concurrent chemotherapy remains unanswered given trials evaluating the use of more contemporary chemotherapy agents is sparse. Although, agents such as bevacizumab and gemcitabine are discouraged (34).
In summary, there is no data which can definitely suggest a benefit to the addition of systemic chemotherapy to palliative radiation. The therapeutic ratio is narrow and the treating Radiation Oncologist should attempt to sequence chemotherapy and radiation therapy as best as possible to provide optimal treatment outcomes with minimal side effects.
ASTRO guidelines for palliative thoracic radiation therapy
ASTRO states short fractionation schedules provide adequate symptomatic alleviation and can be used for patients with poor performance status or those requesting shorter treatment times (34). Higher dose schedules (e.g., 30 Gy in 10 fractions equivalent or greater) may provide a survival benefit for those with a good performance status and is associated with an increase in total symptom score. There is no proven additional benefit to concurrent chemotherapy. There is no concrete randomized evidence to recommend endobronchial brachytherapy with or without other palliative therapies for routine initial palliative management for symptomatic NSCLC tumors. Although, it is a reasonable option as a palliative therapy for previous irradiated areas.
Acknowledgments
Funding: None.
Footnote
Provenance and Peer Review: This article was commissioned by the Guest Editors (Lyudmila Bazhenova and Ajay Pal Singh Sandhu) for the series “Recent advances in radiotherapy and targeted therapies for lung cancer” published in Translational Cancer Research. The article has undergone external peer review.
Conflicts of Interest: All authors have completed the ICMJE uniform disclosure form (available at http://dx.doi.org/10.3978/j.issn.2218-676X.2015.08.03). The series “Recent advances in radiotherapy and targeted therapies for lung cancer” was commissioned by the editorial office without any funding or sponsorship. The authors have no other conflicts of interest to declare.
Ethical Statement: The authors are accountable for all aspects of the work in ensuring that questions related to the accuracy or integrity of any part of the work are appropriately investigated and resolved.
Open Access Statement: This is an Open Access article distributed in accordance with the Creative Commons Attribution-NonCommercial-NoDerivs 4.0 International License (CC BY-NC-ND 4.0), which permits the non-commercial replication and distribution of the article with the strict proviso that no changes or edits are made and the original work is properly cited (including links to both the formal publication through the relevant DOI and the license). See: https://creativecommons.org/licenses/by-nc-nd/4.0/.
References
- Roswit B, Patno ME, Rapp R, et al. The survival of patients with inoperable lung cancer: a large-scale randomized study of radiation therapy versus placebo. Radiology 1968;90:688-97. [PubMed]
- Perez CA, Stanley K, Rubin P, et al. A prospective randomized study of various irradiation doses and fractionation schedules in the treatment of inoperable non-oat-cell carcinoma of the lung. Preliminary report by the Radiation Therapy Oncology Group. Cancer 1980;45:2744-53. [PubMed]
- Perez CA, Pajak TF, Rubin P, et al. Long-term observations of the patterns of failure in patients with unresectable non-oat cell carcinoma of the lung treated with definitive radiotherapy. Report by the Radiation Therapy Oncology Group. Cancer 1987;59:1874-81. [PubMed]
- Perez CA, Bauer M, Edelstein S, et al. Impact of tumor control on survival in carcinoma of the lung treated with irradiation. Int J Radiat Oncol Biol Phys 1986;12:539-47. [PubMed]
- Cox JD, Azarnia N, Byhardt RW, et al. A randomized phase I/II trial of hyperfractionated radiation therapy with total doses of 60.0 Gy to 79.2 Gy: possible survival benefit with greater than or equal to 69.6 Gy in favorable patients with Radiation Therapy Oncology Group stage III non-small-cell lung carcinoma: report of Radiation Therapy Oncology Group 83-11. J Clin Oncol 1990;8:1543-55. [PubMed]
- Saunders M, Dische S, Barrett A, et al. Continuous, hyperfractionated, accelerated radiotherapy (CHART) versus conventional radiotherapy in non-small cell lung cancer: mature data from the randomised multicentre trial. CHART Steering committee. Radiother Oncol 1999;52:137-48. [PubMed]
- Saunders MI, Rojas A, Lyn BE, et al. Experience with dose escalation using CHARTWEL (continuous hyperfractionated accelerated radiotherapy weekend less) in non-small-cell lung cancer. Br J Cancer 1998;78:1323-8. [PubMed]
- Baumann M, Herrmann T, Koch R, et al. Final results of the randomized phase III CHARTWEL-trial (ARO 97-1) comparing hyperfractionated-accelerated versus conventionally fractionated radiotherapy in non-small cell lung cancer (NSCLC). Radiother Oncol 2011;100:76-85. [PubMed]
- Dillman RO, Herndon J, Seagren SL, et al. Improved survival in stage III non-small-cell lung cancer: seven-year follow-up of cancer and leukemia group B (CALGB) 8433 trial. J Natl Cancer Inst 1996;88:1210-5. [PubMed]
- Sause WT, Scott C, Taylor S, et al. Radiation Therapy Oncology Group (RTOG) 88-08 and Eastern Cooperative Oncology Group (ECOG) 4588: preliminary results of a phase III trial in regionally advanced, unresectable non-small-cell lung cancer. J Natl Cancer Inst 1995;87:198-205. [PubMed]
- Furuse K, Fukuoka M, Kawahara M, et al. Phase III study of concurrent versus sequential thoracic radiotherapy in combination with mitomycin, vindesine, and cisplatin in unresectable stage III non-small-cell lung cancer. J Clin Oncol 1999;17:2692-9. [PubMed]
- Curran WJ Jr, Paulus R, Langer CJ, et al. Sequential vs. concurrent chemoradiation for stage III non-small cell lung cancer: randomized phase III trial RTOG 9410. J Natl Cancer Inst 2011;103:1452-60. [PubMed]
- Fournel P, Robinet G, Thomas P, et al. Randomized phase III trial of sequential chemoradiotherapy compared with concurrent chemoradiotherapy in locally advanced non-small-cell lung cancer: Groupe Lyon-Saint-Etienne d'Oncologie Thoracique-Groupe Français de Pneumo-Cancérologie NPC 95-01 Study. J Clin Oncol 2005;23:5910-7. [PubMed]
- Zatloukal P, Petruzelka L, Zemanova M, et al. Concurrent versus sequential chemoradiotherapy with cisplatin and vinorelbine in locally advanced non-small cell lung cancer: a randomized study. Lung Cancer 2004;46:87-98. [PubMed]
- Aupérin A, Le Péchoux C, Rolland E, et al. Meta-analysis of concomitant versus sequential radiochemotherapy in locally advanced non-small-cell lung cancer. J Clin Oncol 2010;28:2181-90. [PubMed]
- Belani CP, Lee JS, Socinski MA, et al. Randomized phase III trial comparing cisplatin-etoposide to carboplatin-paclitaxel in advanced or metastatic non-small cell lung cancer. Ann Oncol 2005;16:1069-75. [PubMed]
- Peters S, Adjei AA. Lung cancer. How much platinum-based chemotherapy is enough in NSCLC? Nat Rev Clin Oncol 2015;12:8-10. [PubMed]
- Belani CP, Ramalingam S, Perry MC, et al. Randomized, phase III study of weekly paclitaxel in combination with carboplatin versus standard every-3-weeks administration of carboplatin and paclitaxel for patients with previously untreated advanced non-small-cell lung cancer. J Clin Oncol 2008;26:468-73. [PubMed]
- Gandara DR, Chansky K, Albain KS, et al. Consolidation docetaxel after concurrent chemoradiotherapy in stage IIIB non-small-cell lung cancer: phase II Southwest Oncology Group Study S9504. J Clin Oncol 2003;21:2004-10. [PubMed]
- Hanna N, Neubauer M, Yiannoutsos C, et al. Phase III study of cisplatin, etoposide, and concurrent chest radiation with or without consolidation docetaxel in patients with inoperable stage III non-small-cell lung cancer: the Hoosier Oncology Group and U.S. Oncology. J Clin Oncol 2008;26:5755-60. [PubMed]
- Zhang C, Huang C, Wang J, et al. Maintenance or Consolidation Therapy for Non-Small-Cell Lung Cancer: A Meta-Analysis Involving 5841 Subjects. Clin Lung Cancer 2015;16:e15-23. [PubMed]
- Albain KS, Swann RS, Rusch VW, et al. Radiotherapy plus chemotherapy with or without surgical resection for stage III non-small-cell lung cancer: a phase III randomised controlled trial. Lancet 2009;374:379-86. [PubMed]
- Andre F, Grunenwald D, Pignon JP, et al. Survival of patients with resected N2 non-small-cell lung cancer: evidence for a subclassification and implications. J Clin Oncol 2000;18:2981-9. [PubMed]
- Machtay M, Lee JH, Stevenson JP, et al. Two commonly used neoadjuvant chemoradiotherapy regimens for locally advanced stage III non-small cell lung carcinoma: long-term results and associations with pathologic response. J Thorac Cardiovasc Surg 2004;127:108-13. [PubMed]
- Socinski MA, Rosenman JG, Halle J, et al. Dose-escalating conformal thoracic radiation therapy with induction and concurrent carboplatin/paclitaxel in unresectable stage IIIA/B nonsmall cell lung carcinoma: a modified phase I/II trial. Cancer 2001;92:1213-23. [PubMed]
- Socinski MA, Morris DE, Halle JS, et al. Induction and concurrent chemotherapy with high-dose thoracic conformal radiation therapy in unresectable stage IIIA and IIIB non-small-cell lung cancer: a dose-escalation phase I trial. J Clin Oncol 2004;22:4341-50. [PubMed]
- Bradley JD, Paulus R, Komaki R, et al. Standard-dose versus high-dose conformal radiotherapy with concurrent and consolidation carboplatin plus paclitaxel with or without cetuximab for patients with stage IIIA or IIIB non-small-cell lung cancer (RTOG 0617): a randomised, two-by-two factorial phase 3 study. Lancet Oncology 2015;16:187-99. [PubMed]
- Faivre-Finn C. Dose escalation in lung cancer: have we gone full circle? Lancet Oncol 2015;16:125-7. [PubMed]
- Belderbos J, Walraven I, van Diessen J, et al. Radiotherapy dose and fractionation for stage III NSCLC. Lancet Oncol 2015;16:e156-7. [PubMed]
- Modelmog D, Rahlenbeck S, Trichopoulos D. Accuracy of death certifi cates: a population-based, complete-coverage, one-year autopsy study in East Germany. Cancer Causes Control 1992;3:541-46. [PubMed]
- Movsas B, Hu C, Sloan J, et al. Quality of Life (QOL) Analysis of the Randomized Radiation (RT) Dose-Escalation NSCLC Trial (RTOG 0617): The Rest of the Story. Int J Radiat Oncol Biol Phys 2013;87:S1-S2.
- Palma DA, Senan S, Tsujino K, et al. Predicting radiation pneumonitis after chemoradiation therapy for lung cancer: an international individual patient data meta-analysis. Int J Radiat Oncol Biol Phys 2013;85:444-50. [PubMed]
- Machtay M, Hsu C, Komaki R, et al. Effect of overall treatment time on outcomes after concurrent chemoradiation for locally advanced non-small-cell lung carcinoma: analysis of the Radiation Therapy Oncology Group (RTOG) experience. Int J Radiat Oncol Biol Phys 2005;63:667-71. [PubMed]
- Rodrigues G, Videtic GM, Sur R, et al. Palliative thoracic radiotherapy in lung cancer: An American Society for Radiation Oncology evidence-based clinical practice guideline. Pract Radiat Oncol 2011;1:60-71. [PubMed]
- Fang LC, Komaki R, Allen P, et al. Comparison of outcomes for patients with medically inoperable Stage I non-small-cell lung cancer treated with two-dimensional vs. three-dimensional radiotherapy. Int J Radiat Oncol Biol Phys 2006;66:108-16. [PubMed]
- Chen AB, Neville BA, Sher DJ, et al. Survival outcomes after radiation therapy for stage III non-small-cell lung cancer after adoption of computed tomography-based simulation. J Clin Oncol 2011;29:2305-11. [PubMed]
- Graham AN, Chan KJ, Pastorino U, et al. Systematic nodal dissection in the intrathoracic staging of patients with non-small cell lung cancer. J Thorac Cardiovasc Surg 1999;117:246-51. [PubMed]
- Kepka L, Maciejewski B, Withers RH. Does incidental irradiation with doses below 50 Gy effectively reduce isolated nodal failures in non-small-cell lung cancer: dose-response relationship. Int J Radiat Oncol Biol Phys 2009;73:1391-6. [PubMed]
- Yuan S, Sun X, Li M, et al. A randomized study of involved-field irradiation versus elective nodal irradiation in combination with concurrent chemotherapy for inoperable stage III nonsmall cell lung cancer. Am J Clin Oncol 2007;30:239-44. [PubMed]
- Giraud P, Antoine M, Larrouy A, et al. Evaluation of microscopic tumor extension in non-small-cell lung cancer for three-dimensional conformal radiotherapy planning. Int J Radiat Oncol Biol Phys 2000;48:1015-24. [PubMed]
- Li WL, Yu JM, Liu GH, et al. A comparative study on radiology and pathology target volume in non-small-cell lung cancer. Zhonghua Zhong Liu Za Zhi 2003;25:566-8. [PubMed]
- De Wever W, Ceyssens S, Mortelmans L, et al. Additional value of PET-CT in the staging of lung cancer: comparison with CT alone, PET alone and visual correlation of PET and CT. Eur Radiol 2007;17:23-32. [PubMed]
- Gould MK, Kuschner WG, Rydzak CE, et al. Test performance of positron emission tomography and computed tomography for mediastinal staging in patients with non-small-cell lung cancer: a meta-analysis. Ann Intern Med 2003;139:879-92. [PubMed]
- Fox JL, Rengan R, O’Meara W, et al. Does registration of PET and planning CT images decrease interobserver and intraobserver variation in delineating tumor volumes for non-small-cell lung cancer? Int J Radiat Oncol Biol Phys 2005;62:70-5. [PubMed]
- Ung YC, Gu C, Cline K, et al. An Ontario Clinical Oncology (OCOG) Randomized Trial (PET START) of FDG PET/CT in Stage 3 Non-small Cell Lung Cancer (NSCLC): Impact of PET on Survival. Int J Radiat Oncol Biol Phys 2011;81:S137.
- Darling GE, Maziak DE, Inculet RI, et al. Positron emission tomography-computed tomography compared with invasive mediastinal staging in non-small cell lung cancer: results of mediastinal staging in the early lung positron emission tomography trial. J Thorac Oncol 2011;6:1367-72. [PubMed]
- Giraud P, Garcia R. Respiratory gating for radiotherapy: main technical aspects and clinical benefits. Bull Cancer 2010;97:847-56. [PubMed]
- Keall PJ, Mageras GS, Balter JM, et al. The management of respiratory motion in radiation oncology report of AAPM Task Group 76. Med Phys 2006;33:3874-900. [PubMed]
- Ling CC, Yorke E, Amols H, et al. High-tech will improve radiotherapy of NSCLC: a hypothesis waiting to be validated. Int J Radiat Oncol Biol Phys 2004;60:3-7. [PubMed]
- Rietzel E, Rosenthal SJ, Gierga DP, et al. Moving targets: detection and tracking of internal organ motion for treatment planning and patient set-up. Radiother Oncol 2004;73:S68-S72. [PubMed]
- Wong JW, Sharpe MB, Jaffray DA, et al. The use of active breathing control (ABC) to reduce margin for breathing motion. Int J Radiat Oncol Biol Phys 1999;44:911-9. [PubMed]
- Remouchamps VM, Letts N, Vicini FA, et al. Initial clinical experience with moderate deep-inspiration breath hold using an active breathing control device in the treatment of patients with left-sided breast cancer using external beam radiation therapy. Int J Radiat Oncol Biol Phys 2003;56:704-15. [PubMed]
- Sixel KE, Aznar MC, Ung YC. Deep inspiration breath hold to reduce irradiated heart volume in breast cancer patients. Int J Radiat Oncol Biol Phys 2001;49:199-204. [PubMed]
- Sharp GC, Jiang SB, Shimizu S, et al. Prediction of respiratory tumour motion for real-time image-guided radiotherapy. Phys Med Biol 2004;49:425-40. [PubMed]
- Murphy MJ. Tracking moving organs in real time. Semin Radiat Oncol 2004;14:91-100. [PubMed]
- Mell LK, Mehrotra AK, Mundt AJ. Intensity-modulated radiation therapy use in the U.S., 2004. Cancer 2005;104:1296-303. [PubMed]
- Wu VW, Kwong DL, Sham JS. Target dose conformity in 3-dimensional conformal radiotherapy and intensity modulated radiotherapy. Radiother Oncol 2004;71:201-6. [PubMed]
- Hong TS, Ritter MA, Tome WA, et al. Intensity-modulated radiation therapy: Emerging cancer treatment technology. Br J Cancer 2005;92:1819-24. [PubMed]
- Liao ZX, Komaki RR, Thames HD Jr, et al. Influence of technologic advances on outcomes in patients with unresectable, locally advanced non-small-cell lung cancer receiving concomitant chemoradiotherapy. Int J Radiat Oncol Biol Phys 2010;76:775-81. [PubMed]
- Kepka L, Bujko K, Zolciak-Siwinska A, et al. Incidental irradiation of mediastinal and hilar lymph node stations during 3D-conformal radiotherapy for non-small cell lung cancer. Acta Oncol 2008;47:954-61. [PubMed]
- Graham MV, Purdy JA, Emami B, et al. Clinical dose-volume histogram analysis for pneumonitis after 3D treatment for non-small cell lung cancer (NSCLC). Int J Radiat Oncol Biol Phys 1999;45:323-9. [PubMed]
- Shen WB, Zhu SC, Gao HM, et al. Low dose volume histogram analysis of the lungs in prediction of acute radiation pneumonitis in patients with esophageal cancer treated with three-dimensional conformal radiotherapy. Zhonghua Zhong Liu Za Zhi 2013;35:45-9. [PubMed]
- Kwa SL, Lebesque JV, Theuws JC, et al. Radiation pneumonitis as a function of mean lung dose: an analysis of pooled data of 540 patients. Int J Radiat Oncol Biol Phys 1998;42:1-9. [PubMed]
- Hernando ML, Marks LB, Bentel GC, et al. Radiation-induced pulmonary toxicity: a dose-volume histogram analysis in 201 patients with lung cancer. Int J Radiat Oncol Biol Phys 2001;51:650-9. [PubMed]
- Shi A, Zhu G, Wu H, et al. Analysis of clinical and dosimetric factors associated with severe acute radiation pneumonitis in patients with locally advanced non-small cell lung cancer treated with concurrent chemotherapy and intensity-modulated radiotherapy. Radiat Oncol 2010;5:35. [PubMed]
- Singh AK, Lockett MA, Bradley JD. Predictors of radiation-induced esophageal toxicity in patients with non-small-cell lung cancer treated with three-dimensional conformal radiotherapy. Int J Radiat Oncol Biol Phys 2003;55:337-41. [PubMed]
- Takeda K, Nemoto K, Saito H, et al. Dosimetric correlations of acute esophagitis in lung cancer patients treated with radiotherapy. Int J Radiat Oncol Biol Phys 2005;62:626-9. [PubMed]
- Werner-Wasik M, Yorke E, Deasy J, et al. Radiation dose-volume effects in the esophagus. Int J Radiat Oncol Biol Phys 2010;76:S86-93. [PubMed]
- Hardy D, Liu CC, Cormier JN, et al. Cardiac toxicity in association with chemotherapy and radiation therapy in a large cohort of older patients with non-small-cell lung cancer. Ann Oncol 2010;21:1825-33. [PubMed]
- Gagliardi G, Constine LS, Moiseenko V, et al. Radiation dose-volume effects in the heart. Int J Radiat Oncol Biol Phys 2010;76:S77-85. [PubMed]
- Kong FM, Frey KA, Quint LE, et al. A pilot study of [18F]fluorodeoxyglucose positron emission tomography scans during and after radiation-based therapy in patients with non small-cell lung cancer. J Clin Oncol 2007;25:3116-23. [PubMed]
- Feng M, Kong FM, Gross M, et al. Using fluorodeoxyglucose positron emission tomography to assess tumor volume during radiotherapy for non-small-cell lung cancer and its potential impact on adaptive dose escalation and normal tissue sparing. Int J Radiat Oncol Biol Phys 2009;73:1228-34. [PubMed]
- Lin Q, Liu YE, Ren XC, et al. Dose escalation of accelerated hypofractionated three-dimensional conformal radiotherapy (at 3 Gy/fraction) with concurrent vinorelbine and carboplatin chemotherapy in unresectable stage III non-small-cell lung cancer: a phase I trial. Radiat Oncol 2013;8:201. [PubMed]
- Mehta M, Scrimger R, Mackie R, et al. A new approach to dose escalation in non-small-cell lung cancer. Int J Radiat Oncol Biol Phys 2001;49:23-33. [PubMed]
- Thirion P, Holmberg O, Collins CD, et al. Escalated dose for non-small-cell lung cancer with accelerated hypofractionated three-dimensional conformal radiation therapy. Radiother Oncol 2004;71:163-6. [PubMed]
- Uitterhoeve AL, Belderbos JS, Koolen MG, et al. Toxicity of high-dose radiotherapy combined with daily cisplatin in non-small cell lung cancer: results of the EORTC 08912 phase I/II study. European Organization for Research and Treatment of Cancer. Eur J Cancer 2000;36:592-600. [PubMed]
- Belderbos J, Uitterhoeve L, van Zandwijk N, et al. Randomised trial of sequential versus concurrent chemo-radiotherapy in patients with inoperable non-small cell lung cancer (EORTC 08972-22973). Eur J Cancer 2007;43:114-21. [PubMed]
- Maguire J, Khan I, McMenemin R, et al. SOCCAR: A randomised phase II trial comparing sequential versus concurrent chemotherapy and radical hypofractionated radiotherapy in patients with inoperable stage III Non-Small Cell Lung Cancer and good performance status. Eur J Cancer 2014;50:2939-49. [PubMed]
- Rowell NP, Gleeson FV. Steroids, radiotherapy, chemotherapy and stents for superior vena caval obstruction in carcinoma of the bronchus: a systematic review. Clin Oncol (R Coll Radiol) 2002;14:338-51. [PubMed]
- Lester JF, Macbeth FR, Toy E, et al. Palliative radiotherapy regimens for non-small cell lung cancer. Cochrane Database Syst Rev 2006;CD002143 [PubMed]
- Budach W, Belka C. Palliative percutaneous radiotherapy in non-small-cell lung cancer. Lung cancer 2004;45:S239-45. [PubMed]
- Toy E, Macbeth F, Coles B, et al. Palliative thoracic radiotherapy for non-small-cell lung cancer: a systematic review. Am J Clin Oncol 2003;26:112-20. [PubMed]
- Kramer GW, Wanders SL, Noordijk EM, et al. Results of the Dutch National study of the palliative effect of irradiation using two different treatment schemes for non-small cell lung cancer. J Clin Oncol 2005;23:2962-70. [PubMed]
- Teo P, Tai TH, Choy D, et al. A randomized study on palliative radiation therapy for inoperable non small cell carcinoma of the lung. Int J Radiat Oncol Biol Phys 1988;14:867-71. [PubMed]
- Mallick I, Sharma SC, Behera D, et al. Optimization of dose and fractionation of endobronchial brachytherapy with or without external radiation in the palliative management of non-small cell lung cancer: a prospective randomized study. J Cancer Res Ther 2006;2:119-25. [PubMed]
- Stout R, Barber P, Burt P, et al. Clinical and quality of life outcomes in the first United Kingdom randomized trial of endobronchial brachytherapy (intraluminal radiotherapy) vs. external beam radiotherapy in the palliative treatment of inoperable non-small cell lung cancer. Radiother Oncol 2000;56:323-7. [PubMed]
- Huber RM, Fischer R, Haŭtmann H, et al. Palliative endobronchial brachytherapy for central lung tumors. A prospective, randomized comparison of two fractionation schedules. Chest 1995;107:463-70. [PubMed]
- Ung YC, Yu E, Falkson C, et al. The role of high-dose-rate brachytherapy in the palliation of symptoms in patients with non-small cell lung cancer: a systematic review. Brachytherapy 2006;5:189-202. [PubMed]
- Michael M, Wirth A, Ball DL, et al. A phase I trial of high-dose palliative radiotherapy plus concurrent weekly vinorelbine and cisplatin in patients with locally advanced and metastatic NSCLC. Br J Cancer 2005;93:652-61. [PubMed]
- Hoffman PC, Cohen EE, Masters GA, et al. Carboplatin plus vinorelbine with concomitant radiation therapy in advanced non-small cell lung cancer: a phase I study. Lung Cancer 2002;38:65-71. [PubMed]
- Jeremic B, Shibamoto Y, Milicic B, et al. Short-term chemotherapy and palliative radiotherapy for elderly patients with stage IV non-small cell lung cancer: a phase II study. Lung Cancer 1999;24:1-9. [PubMed]
- Ball D, Smith J, Bishop J, et al. A phase III study of radiotherapy with and without continuous-infusion fluorouracil as palliation for non-small-cell lung cancer. Br J Cancer 1997;75:690-7. [PubMed]